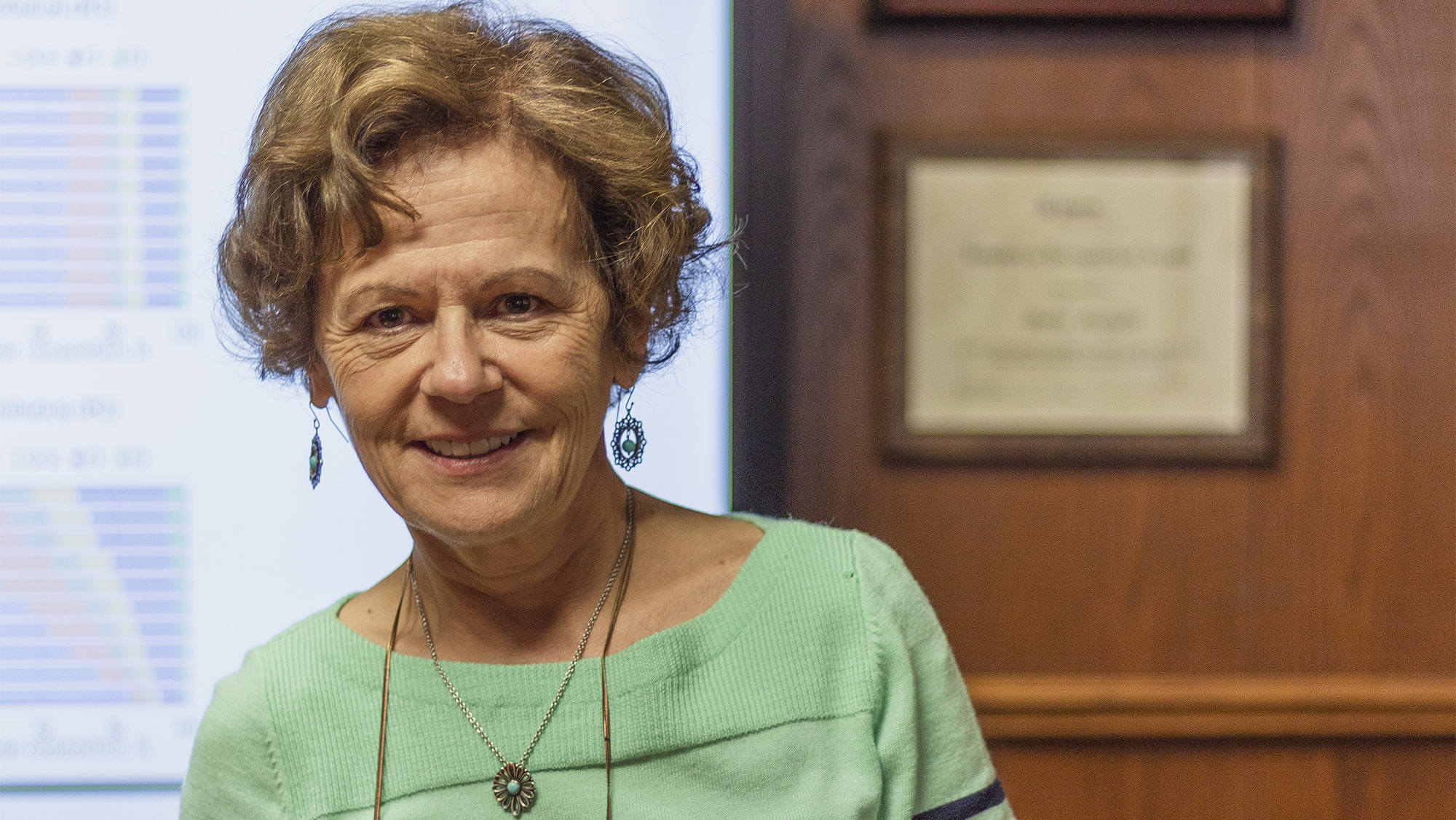
Researchers at Texas A&M University have found that the presence of a fossilized organic substance called kerogen plays a vital role in how easily carbon dioxide can travel through shale reservoirs.
Why is this important?
Injecting carbon dioxide into shale reservoirs accomplishes two critical results. The action stores an unwanted greenhouse gas safely underground and also improves the flow of oil and gas within the shale rock for recovery by the petroleum industry. However, less carbon dioxide is going into these reservoirs for storage, and the massive quantity of oil and gas recovered is still far lower than predicted.
“Models used to predict carbon dioxide movement through formations are inaccurate because most simulations are focused on limited information: the properties of shale,” said Dr. Maria Barrufet, a professor in the Harold Vance Department of Petroleum Engineering. “We need a realistic forecast that includes carbon dioxide interaction with reservoir fluids, materials and environments. We cannot neglect the other facets in the mix.”
Shale reservoirs are mainly formed with layered shale, a dense rock containing tiny pores similar in diameter to a human hair. Sandwiched between these layers, often hundreds of miles wide, are millions of pockets of inorganic minerals such as clay, quartz and pyrite, along with an organic mineral called kerogen. Since shale properties are relatively well known, Barrufet and her team considered this complex combination of inorganic and organic materials when tackling the mystery of reduced storage and flow.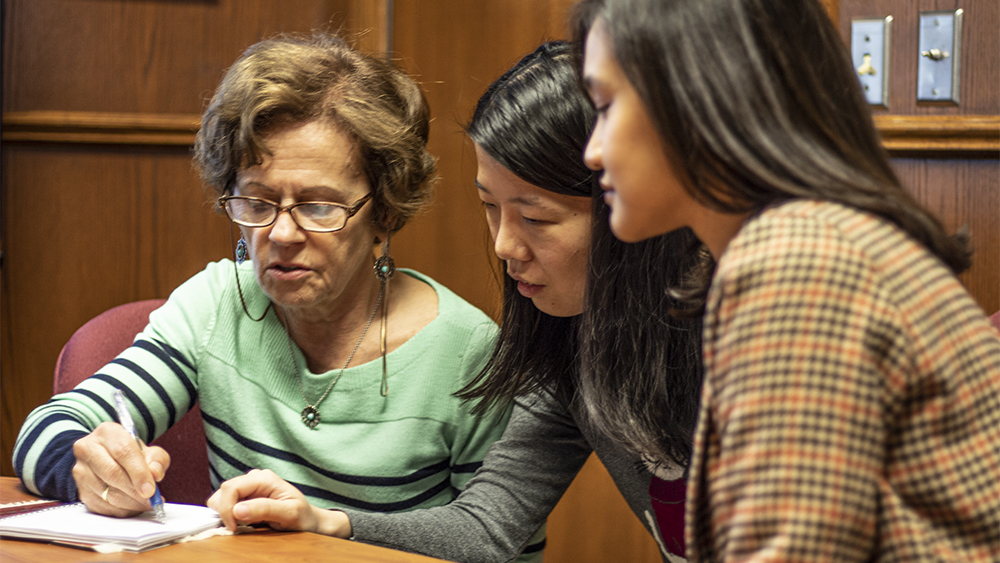
Results of their lab tests, published online in Applied Energy, showed that kerogen could not absorb carbon dioxide quickly, so it reduced the effectiveness of injection processes.
Kerogen is an abundant source of fuel, from its first stage of development as loosely packed peat on the Earth’s surface to deep underground where it bakes into its final stage as solidified coal. In a shale reservoir, kerogen is compressed by the layered rock and slowly oozes out oil molecules that seep into the surrounding shale and inorganic minerals. Petroleum companies fracture the dense shale to create channels where the oil can flow. Still, fracturing alone does not always speed recovery. Oil molecules react to the pressure changes and temperatures within the reservoir and can switch from a liquid state to a gas state and back again. This phase change behavior hampers flow.
“Unlike conventional reservoirs where rock pore sizes easily allow fluid flow, pores sizes in shale are only slightly larger than the oil molecules,” Barrufet said. “These confined molecules feel like prisoners and want to escape. If you fracture a space containing these trapped molecules, you suddenly change the pressure from high to low in that space. There will be a rush for the exit as the pressure releases, but the two phases, liquid and gas together, will compete with each other and block the door.”
Over the years, testing with shale core samples showed that carbon dioxide would move quickly in fractured shale rock. When injected, carbon dioxide improves the flow of oil and gas by pushing these molecules out of the rock and into fracture channels. However, not much attention was paid to how well carbon dioxide traveled through organics like kerogen.
Barrufet’s research team used shale core samples, cut pieces of rock one and a half inches in diameter, and approximately six inches tall for their experiments. Pockets of kerogen were embedded in the center of these cores before the samples were saturated with oil. The cores were inserted in tubes packed with glass beads to simulate fractured shale channels and then injected with carbon dioxide using the two leading industry methods: core flooding and huff and puff.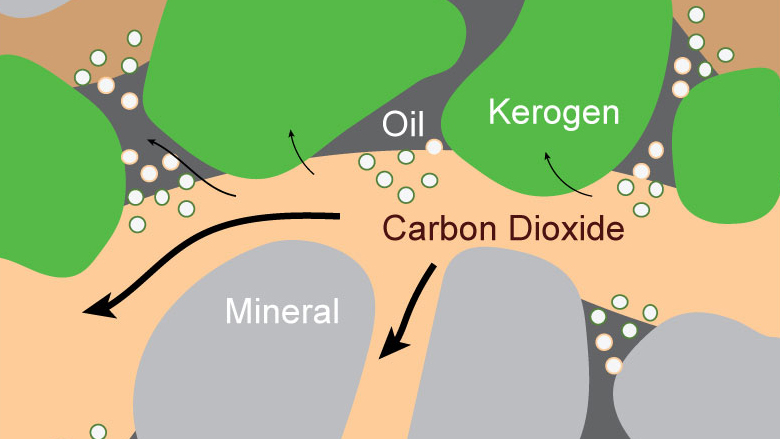
Modeling the lab experiment results found a critical behavior pattern: oil-saturated organic matter acted as a barrier. That is, the kerogen would not allow a high-pressure application of carbon dioxide to move through it easily. Yet, results also showed that when pressures were kept up over an extended time, the kerogen slowly absorbed the greenhouse gas.
“It is the difference of ‘huff and puff’ to ‘huff and huff,’” Barrufet explained. “In many field applications, carbon dioxide is injected, a ‘huff,’ and then pressure is reduced, a ‘puff,’ to allow production to resume. A continuous pressure injection, known as core flooding, is a ‘huff and huff.’ But there also needs to be an extended soak time under this pressure, to take advantage of the slow fluid movement within kerogen. A waiting period allows the organic minerals to soak up the injected gas, which eventually displaces the oil and increases recovery.”
Kerogen pockets can form clusters that span significant areas of space. Barrufet and her team noted that high injection pressures alone do not allow as much storage of carbon dioxide or recovery of oil and gas because the kerogen prevents carbon dioxide from reaching large quantities of trapped oil. Continuous pressure held for an extended time allowed far greater movement of carbon dioxide through kerogen and into the spaces and materials beyond it, producing better storage and more substantial recoveries.
“It is a fine, delicate balance,” said Barrufet. “Injections are critical to recovery and storage, but work best if applied with an understanding of the complexities within the reservoir. As a next step, we’d like to do field trials to confirm our results better.”