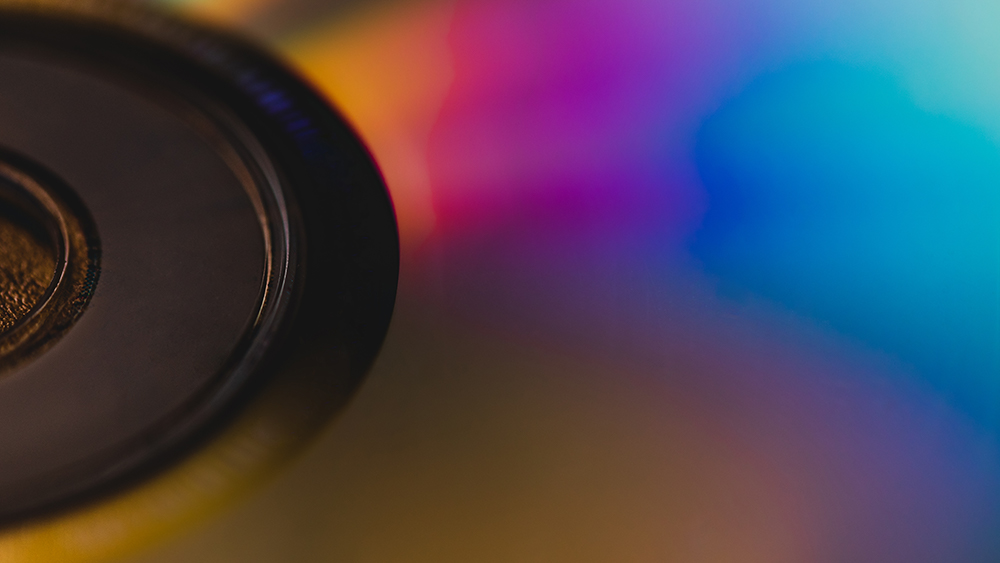
If you have witnessed the rainbow pattern that dances on the surface of a CD or DVD, then you have seen diffraction at work. The disk acts as a diffraction grating, an optical element that disperses light into various colors or wavelengths. This division of light can occur on any periodic, or rippled, surface. The direction of these divided light beams, and subsequent scattering of light, can be estimated through a commonly used set of equations called the nonparaxial scalar diffraction theory.
Dr. Christi Madsen, professor in the Department of Electrical and Computer Engineering at Texas A&M University, is testing the limits of this fundamental theory so that a more accurate understanding of scattering losses can be achieved. Madsen is working to improve the systems that generate solar power through the use of concentrating mirrors or lenses by getting the light more efficiently to the converter — whether photovoltaic, which converts sunlight into electricity, or thermal, which converts heat to electricity — and reducing the overall system loss.
Her research on the topic was published in the March issue of the journal Applied Optics. With this paper, Madsen addressed how far off a basic diffraction efficiency calculation could be (easily over 10%) on estimating scattering efficiency and then showed how to get within 1-2% accuracy on the calculation.
An optical fiber is a flexible, transparent fiber that transmits light between the two ends. Picture a light-up toy with clear, transparent fibers that might be purchased for a child to wave around at a concert or event. Madsen explained that standard optical fibers are useful for many real-world applications, like computer networking and telecommunications, but they simply are not practical to relocate sunlight to another location due to their small size.
Because of the limited brightness of solar light compared to lasers, larger waveguides, or light pipes, must be used to transport the concentrated sunlight from point A to point B.
“Light pipes are large versions of optical fibers, which carry light extremely long distances with very low loss (e.g., greater than 90% transmission efficiency over a mile distance) but have a very tiny area that guides the light (e.g., 10-micron diameter, compared to 1 millimeter or larger for light pipes),” Madsen said.
Though light pipes are promising, particularly when made from glass, they currently suffer higher losses during the transmission of light due to scattering at the surface, which is a significant technological issue – one Madsen is determined to change.
“One of the dominant losses occurs at the surface of the waveguide,” Madsen said. “So, if we can get those scattering losses low — as low as in an optical fiber — we could go a long distance with the concentrated sunlight.”
Instead of converting solar light into electrical power for immediate use, Madsen envisions remoting light energy to another location optically by concentrating solar light and using waveguides.
With 1 kilowatt per square meter from the sun, concentration factors on the order of 1,000 enable significant amounts of solar power to be transmitted over light pipes to a separate location and then converted to thermal or electrical energy. An example is industrial process heating, where manufacturing processes are remotely located from the solar collection area. Light pipes have the potential to transport optical power with higher efficiency than the heat transfer fluid systems used currently.
Madsen's next steps will be to determine how closely measurements on fabricated light pipes and simulations are which will provide her an accurate idea of the surface quality required for a given light pipe transmission versus length.