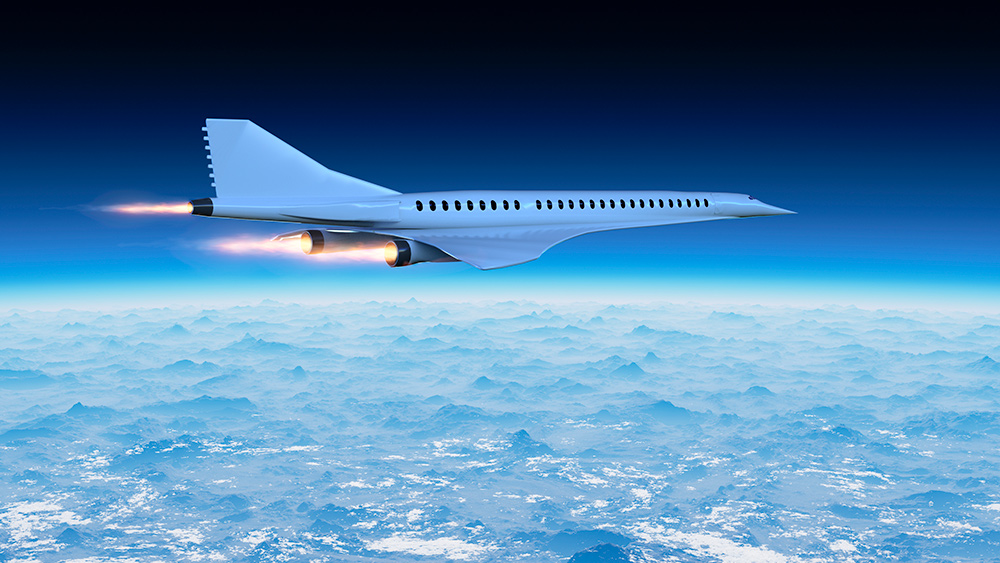
Hypersonic technology presents a vast number of revolutionary opportunities, including the advancement of future commercial flight travel with the potential to reach speeds of 4,000 miles per hour — nearly eight times faster than current average cruising speeds for commercial airliners.
Before implementation, however, researchers must ensure the vessels themselves can withstand the challenges of traveling at such high speeds. One hazard for next-generation hypersonic vessels is hydrometeors — water or ice particles that have formed in the atmosphere, including clouds, fog, snow, rain or hail — which have the potential to cause significant damage to the vehicle structure at hypersonic speeds.
The U.S. Office of Naval Research is supporting a research team led by Dr. Dorrin Jarrahbashi in better understanding the impacts of these hydrometeors, as well as solid particles like ash and dust, on hypersonic vessels upon their re-entry to the atmosphere.
“The goal of this research is to enhance the fundamental understanding of the evolution of aero breakup and evaporation of rain droplets and the behavior of ice particles approaching the transient shocked flow induced by a hypersonic projectile flying through precipitating clouds,” said Jarrahbashi, assistant professor in the J. Mike Walker '66 Department of Mechanical Engineering at Texas A&M University.
If successful, the research will be able to better estimate the physical damage on a hypersonic vessel by predicting the state of the particles and flowfield around it.
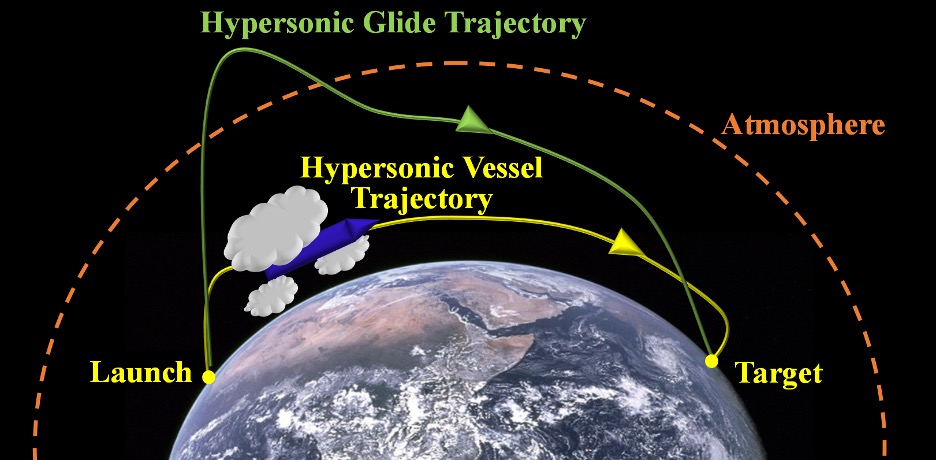
While each flight is different — with its potential for encountering hazards determined in part by its objectives and flight trajectory — takeoff and landing pose the greatest likelihood for a hypersonic vessel to encounter hydrometeors, which could present a significant erosion hazard to its thermal protection system or even cause the nose-tip to change shape. Either outcome has the potential to set off a cascade of other damaging effects for the vessel.
“A change in the nose shape and enhanced surface roughness affect the flowfield, causing extra heating and shear to the vehicle body that ultimately controls the vehicle’s aerodynamic performance,” Jarrahbashi said. “The next generation of hypersonic projectiles requires novel component design and resilient materials to withstand the impact of hydrometeors.”
To aid in developing better designs and materials that are adequately equipped to handle these particles, Jarrahbashi and her team are set to apply a comprehensive computational framework to address this versatility in scales that are currently lacking without the availability of practical experimentation. The team will also validate their findings through shock tube measurements in collaboration with the Fluid Mixing at Extreme Conditions Laboratory directed by Dr. Jacob McFarland, associate professor of mechanical engineering.
The team specifically hopes to augment a material damage model extensively used by the U.S. Department of Defense by adding adjustments to account for expected particulate flow behavior when traveling at hypersonic speeds.
“The attributes of particle clouds — and particularly ice crystals — obtained in this project can be used in surface erosion models to estimate the extent of the damage to the hypersonic vessel,” Jarrahbashi said. “Understanding the effect of the shock layer to decelerate and deflect particles to protect the material of hypersonic vehicles is a key step to predict the resistance of different materials and the potential influence of the shocked particulate flows on the vehicle designs.”
The advancements planned for this project will provide direct contributions to the improvement of the performance and resilience of current and future U.S. Navy hypersonic technologies.