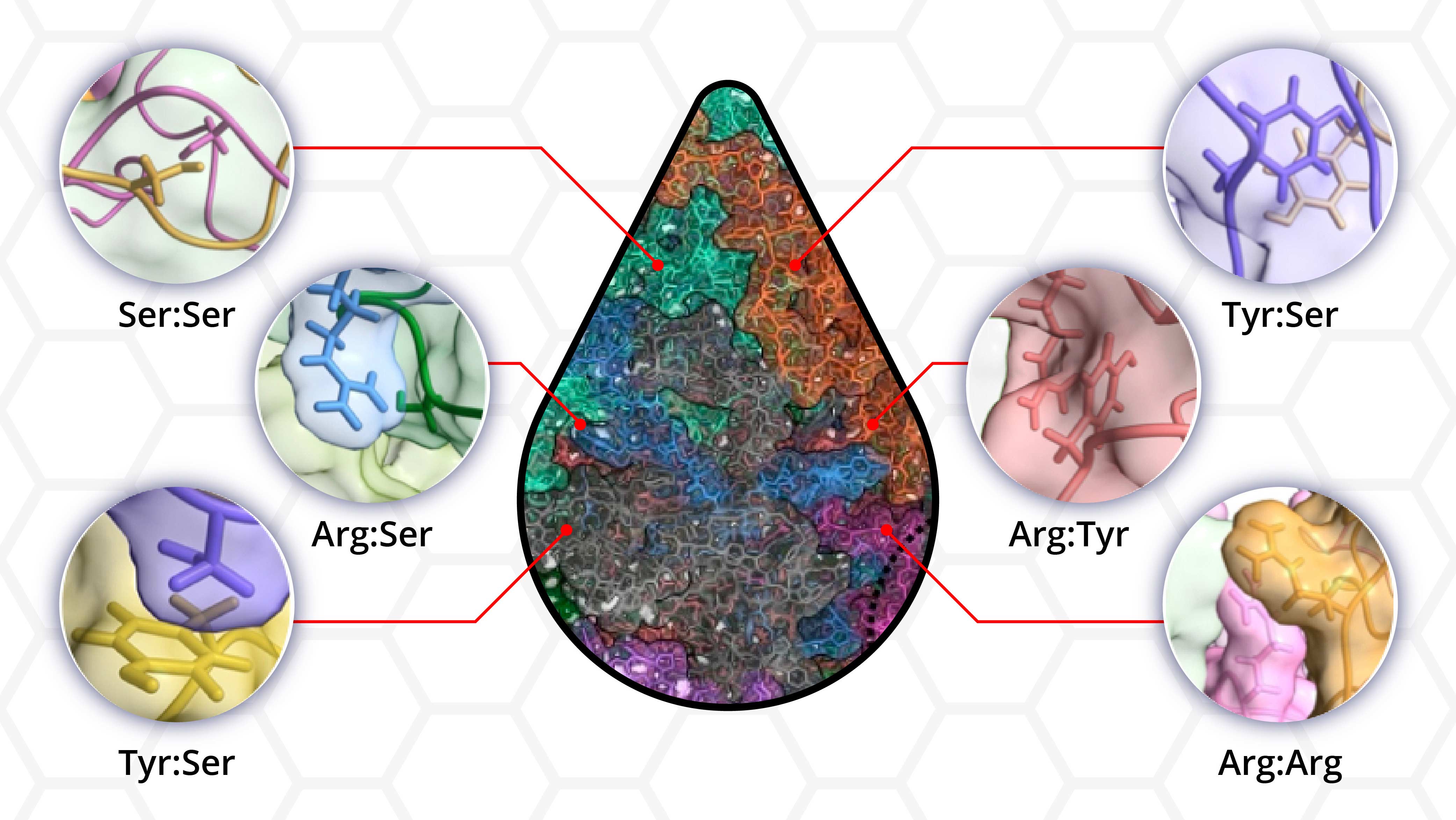
Peering into a biological cell reveals a bustling microscopic world. The workhorses within this realm are specialized structures called organelles that perform vital cellular functions. Curiously, some organelles defy accepted convention: instead of being enclosed within a protective membrane, they are without membranes and take the form of isolated liquid droplets. The rules that govern the formation of these droplets, a process called “liquid-liquid phase separation,” is a new and hotly pursued area of research.
A team of scientists from Texas A&M Engineering, the University of Delaware and Rutgers University have uncovered that amino acids, or residues, making up the proteins within droplets interact in many more ways than is currently recognized. These interactions, they show, facilitate protein assembly and, eventually, liquid-liquid phase separation into droplets.
The researchers have published their findings in the journal Nature Chemistry.
Their work is a step toward broadening the understanding of cellular biology, developing treatments for diseases involving pathologic protein aggregates, like Alzheimer’s and Parkinson’s, and engineering novel bioengineered soft materials.
The recent discovery of liquid droplets within living cells was first made in the germ cells of a soil-dwelling worm, Caenorhabditis elegans (C. elegans). Within the worm’s embryo, membrane-less structures called P granules serve essential reproductive functions. When probed further, investigators found that the P granules lacked membranes and could drip, join together or dissolve away, having characteristics just like liquids. Further, these P granules could hold their integrity within the jelly-like cytoplasm, much like oil droplets in water.
Their work is a step toward broadening the understanding of cellular biology, developing treatments for diseases involving pathologic protein aggregates, like Alzheimer’s and Parkinson’s, and engineering novel bioengineered soft materials.
“There was a fundamental change in 2009 in thinking about cellular compartmentalization in terms of the emergence of droplet-like structures,” said Dr. Jeetain Mittal, professor in the Artie McFerrin Department of Chemical Engineering and senior author. “Most biologists began to accept that phase separation is not the exception but the rule with which biological cells compartmentalize functional units other than membrane-bound organelles.”
But how do only specific proteins squiggling around in the cytoplasm alongside millions of others assemble into functional droplets? Evidence indicates that intrinsically disordered proteins or those lacking an ordered three-dimensional structure may be essential in phase separation. However, the interactions between disordered proteins orchestrating phase separation have yet to be fully delineated.
“We still don’t have a very clear idea of which amino acids within the disordered regions provide the driving force for phase separation,” said Shiv Rekhi, a graduate student in Mittal’s laboratory and lead author. “We wanted to go outside of established rules, still show phase separation, and then quantify how each amino acid contributed to the process.”
For their research, the team used a synthetic disordered protein with amino acid sequences reminiscent of naturally occurring proteins. The researchers then created protein variants by removing or adding a specific type of amino acid and evaluated if condensation to droplets still occurred. With their collaborators, they performed microscopy and turbidity experiments to evaluate the physical nature of the protein-enriched droplet. Finally, using large-scale simulations, Rekhi explored how the atomic interactions between the amino acids within the protein sequence translated to the formation of liquid droplets observed experimentally.
This and many other such experiments told us phase separation can occur without many residues people think are necessary.
“A prevalent view is that tyrosine and/or arginine are required for phase separation. We tested that directly by making protein variants where we removed these residues, and we still got phase separation,” said Rekhi. “This and many other such experiments told us phase separation can occur without many residues people think are necessary.”
The researchers found that all but one of the 12 protein variants showed phase separation, underscoring the presence of multiple interactions between the amino acid residues making up the disordered protein.
“For a while, people in the field have assumed that a limited set of rules can describe droplet formation. We have shown that everything in the protein sequence matters,” said Mittal. “Our paper establishes that the molecular language of phase separation is much richer and more complex.”
Other contributors to the research include Cristobal Garcia Garcia and Dr. Kristi L. Kiick from the University of Delaware; Mayur Barai and Dr. Benjamin Schuster from Rutgers, the State University of New Jersey.
This research is funded by the National Science Foundation, the National Institute of General Medical Science of the National Institute of Health and the Welch Foundation.